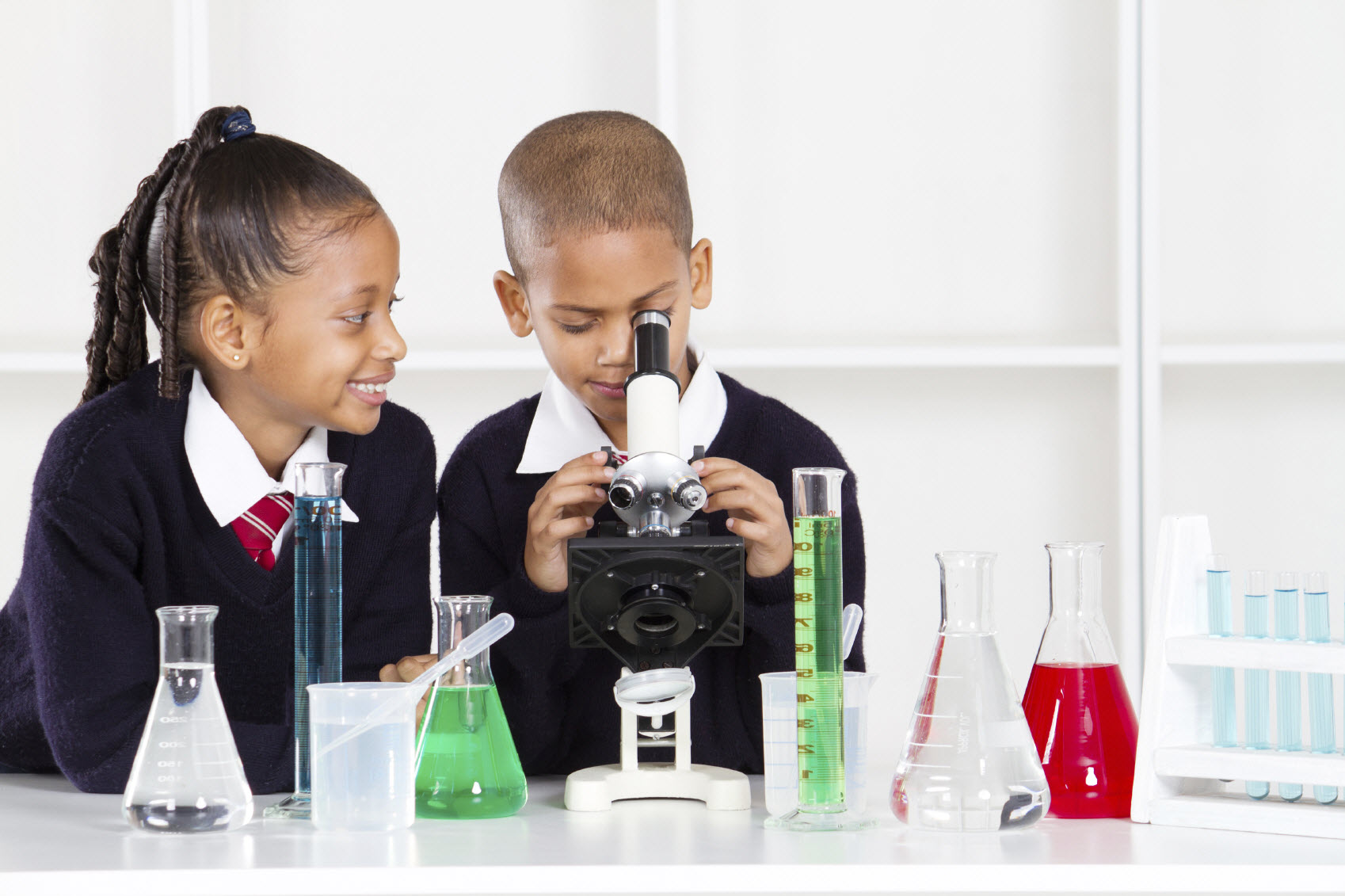
You’ve probably heard the dire news: American kids are falling behind the rest of the world in STEM (science, technology, engineering, and math) education. STEM jobs are going unfilled because there aren’t enough qualified applicants. The U.S. has a STEM shortage; a STEM problem.
But what does it all mean, in real terms, for your child’s education? What is a STEM job, where is the shortage; and how does the need for these workers trickle down to the curriculum taught in your own school?
To begin with, what exactly are these jobs we keep hearing about?
STEM jobs today and tomorrow
A spokesperson for the National Institutes of Health says that for the NIH to accomplish its mission it must either hire or support through grants “a surprising variety of STEM specialties.” The NIH “uses all of the scientific specialties you might imagine being involved in medical research (doctors, nurses, microbiologists, geneticists, etc.) and also many specialties you might not imagine (mathematicians, physicists, computer scientists, medical artists).”
“If a biologist gets a job in a cancer lab, I’d say that’s a STEM job, even though that person may not spend much time doing mathematics or engineering,” says Raymond Johnson, a doctoral student in mathematics education at the University of Colorado in Boulder. “Likewise, someone who studies ergonomic design might be doing a STEM job, even thought there might be no chemistry involved.” Looking at STEM as its own field, “the intersection of a set of activities and skills that we previously considered as distinct,” instead of relying on our historical understanding of specific jobs and disciplines, “is where STEM education gets exciting,” he says.
“Just about every career, from law enforcement to health care, includes a lot more STEM than before,” says Mark Grayson, executive director of STEM learning at Six Red Marbles, an educational publishing and learning design company based in Baltimore.
Emphasizing the need for early, excellent STEM education, he reiterates the point that it’s not about drilling kids in facts. By the time kids start working, Grayson says they’ll need to use their education to “solve a problem that didn’t exist when they went to school. Some of the fastest-growing (and best-paying) careers in the next few decades are expected to be in fields like biomedical engineering, software development and medical technology.”
Linda P. Rosen, CEO of Change the Equation (CTEq), an organization dedicated to translating STEM needs into educational action, whose members include major corporations like 3M, Microsoft, and Xerox, says that the organization’s definition of a STEM-specific job includes computer, architecture, engineering, and physical science occupations, but also “healthcare and management occupations that require strong STEM skills,” such as information systems managers or healthcare practitioners.
“The fastest-growing fields,” she notes, “are also the fields reporting the greatest shortages. Many CTEq companies report a shortage of engineers, often those with specialized training. Companies that require security clearance — which at a minimum requires U.S. citizenship — report STEM shortages at all education levels.”
With the U.S. ranked 52nd in STEM education, and Americans losing interest in pursuing these fields, it really does look like our kids’ education is not preparing them for the future.
Bridging the gap
Answering the question of why American kids aren’t ending up in STEM fields is more complex than you might think.
“First, most schools do nothing with the technology and engineering parts of STEM,” says Mark Grayson. “Second, the learning model is very passive and based on regurgitation of facts.” This is opposed to what he calls a more active model, which emphasizes critical thinking and “the ability to figure out what you need to teach yourself to solve a real-world problem.” Learning passively also “gives math and science an undeserved reputation of being boring and hard.”
It’s not only poor curricula in individual subjects, says Raymond Johnson, but the lack of hands-on experience and a failure to integrate individual STEM disciplines, which would require different models both of teacher preparation and how schools are structured. The best model he’s seen, he says, is the Nature, Life, Technology (NLT) curriculum from the Netherlands. “The samples I’ve seen are fascinating, integrating physics, mathematics, earth science, chemistry, and other disciplines. But most high schools and middle schools in the U.S. don’t have that kind of schedule flexibility, nor do we have teachers with expertise across STEM disciplines.”
Mark Grayson agrees, bringing up compartmentalization as a problem in the current school model.
“Math instruction is over here in this room with this teacher, science is handled over there with that teacher, and rarely do the two meet. Even within science, biology is one thing and physics is another.”
Maria Zacharias of the National Science Foundation points to the work of Robert Tai at the University of Virginia, whose extensive research in K-12 STEM education highlights the importance of early exposure to science. Tai’s best-known longitudinal study tracked students from eighth grade through graduate school and found that, in many STEM fields, students’ early interest in science led them to careers or graduate work in those fields. “If they form interest [by eighth grade],” says Zacharias, “they are more likely to be scientists. If not, it’s hard to get them back.”
Building skills and momentum
The other side of the equation is the lack of in-depth knowledge and training, what some call rigorous learning.
“A good learning experience should make you think, and teach you something you’ll remember and use again,” says Grayson. “The word ‘rigor’ sums this up.”
But rigor is not the only way to describe the quality of educational activities, says Raymond Johnson. ‘Authentic"might be a better choice. “When students do laboratory work to test their own understanding about how something works, and to refine their knowledge, then we’re more closely resembling the work of professional scientists.”
Another way to gauge quality is to think about “cognitive level” or “cognitive demand,” which requires students to “do the mental ‘heavy lifting’ of evaluating a problem and choosing their own solution strategy.”
Giving students a deep bedrock of scientific experience is crucial because early interest, even passion, isn’t always enough. What very few people are willing to admit is that actual scientific work can be tedious and boring.
The onus is on educators and parents to ensure enthusiasm and deep engagement so that later, repetitive work won’t deter students from pursuing STEM fields.
Engineering a working curriculum
How does all of this translate to the classroom?
“At the elementary age,” says Grayson, “a great thing to do is to link engineering with play. Who can build a bridge out of blocks that will hold the most weight? Who can make the paper airplane that flies the fastest or the highest or the farthest? Who can explain why that bridge or airplane worked? Who can figure out how to make one that’s even better?”
Penny Dowdy, a curriculum specialist at Six Red Marbles, demonstrates the kind of deep learning that can happen through just one fourth-grade Common Core mathematics standard.
“A teacher could provide local bus schedules and have students calculate how long it would take to get between destinations, time of arrival, what time they should leave one location to make a transfer, distance traveled, etc. This would hit the ‘use operations to solve word problems involving distances, intervals of time …’ The teacher could provide specific word problems to solve, and then he or she could have students develop their own problems by planning bus trips to destinations that interest them.”
Crucially, Dowdy says, “the activity itself gives students a reason to use math in a practical, real-world way so students aren’t wondering when they would ever use these skills.”
At older ages, the question becomes how to maintain momentum in these areas, and provide a solid foundation for scientific practice and methods.
“By high school,” says Mark Grayson, “a project can involve solving science and engineering challenges in the community using hand-held data recorders. This allows for the learning to link to social studies and other fields as well. Examples include testing local watersheds, monitoring migrating butterflies, identifying acoustic ‘dead spots’ in the auditorium, or analyzing the hot and cold spots in a building.”
The STEM School (recently renamed the Nikola Tesla STEM High School ) in Redmond’s Lake Washington school district has taken this kind of learning to heart, enrolling its students in internships to “tackle real-world problems.” One group recently presented work on a solar light tower project they completed with the guidance of engineers from Genie, a company that manufactures equipment for the construction industry.
Raymond Johnson also sees that some of the solutions to our STEM problems might be found in skills and practices that have been devalued over the last few decades. When he worked as a teacher, he taught at one school “with a very successful construction program in which, each year, the students would build a house.” The sale of the house paid for the program, plus the graduates were prepared for employment in construction work.
“The STEM skills and occupations of the future may look different, but I think we can learn from these kinds of apprenticeship models of education that have worked well with skilled trades.”
The future of STEM education will ideally bring together all of these practices, crossing curricular boundaries to tap into young students’ thirst for exploration, allowing plenty of time for in-depth learning, and creating opportunities for increased hands-on practice. In that space we’ll find our future engineers, mathematicians, biologists, programmers, inventors, and so much more.
Learn more
There is a dizzying array of programs all over the country dedicated to improving both the quality and experience of U.S. STEM education.
The NIH’s Science Education Partnerships (SEPA) grant program currently supports 67 active projects nationwide, such as Seattle-based BioQuest, which offers half-day programs and internships for high-schoolers interested in learning about global health or medical research. The SEPA website allows you to easily search for programs by state.
The NIH also provides curriculum supplements for teachers on a wide array of subjects.
Change the Equation’s STEMworks programs seek to encourage active, hands-on learning at all grade levels.
Washington STEM drives innovation and improvement in STEM education by investing in teacher training, building networks of STEM professionals and educators and advocating for important policy changes.